Guest article
How next-generation sequencing will impact your food safety program
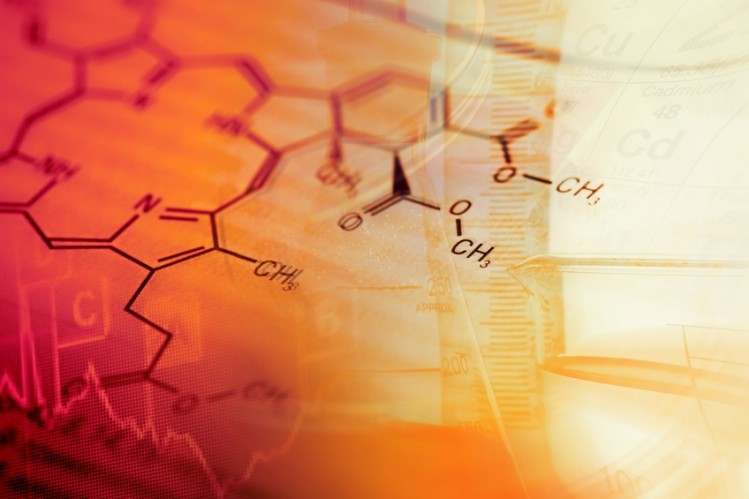
To get a better idea, let’s dig into three different applications of NGS and how they are impacting food safety.
But first, here’s a quick definition of NGS.
What Is NGS, and how did we get here?
In 1885, Theodor Escherich discovered E. coli in the human colon. In that same year, Theobald Smith and David E. Salmon found the first strain of Salmonella. Since then, our knowledge of foodborne pathogens has increased drastically, but detection methods have been slow to change. Cell culturing, a methodology with its roots in the nineteenth century, still remains the gold standard for confirmation in the food safety industry.
Of course, there have been advances along the way, chief of those being the polymerase chain reaction (PCR) technique. In the 1980s, Kary B. Mullis invented the technique that allowed scientists to make numerous copies of a small amount of DNA. Then, in the mid-1990s, two commercial real-time PCR instruments reached the market. To date, PCR testing remains the industry standard for pathogen detection despite the technique’s shortcomings, including high rates of false negatives and positives, as well as binary yes/no answers.
Recognizing the limitations of PCR, more and more food safety practitioners are turning to NGS. In fact, two-thirds of European countries are using whole-genome sequencing (WGS), one of several applications of NGS, for national surveillance of human pathogens.
So, what is NGS? In short, next-generation sequencing (NGS) is the overarching descriptor for sequencing millions of DNA data points at the same time. With so much data at their fingertips, scientists can learn more about food and environmental microbial communities than ever before. Food safety professionals no longer have to ask, “Is this specific microbe in my sample?” Instead, they can ask, “What is the complete microbial makeup of my sample?” That is a powerful shift.
There are several ways to determine the microbial makeup of a sample with NGS. Let’s look at three of the most applicable applications for food safety: shotgun metagenomics sequencing, WGS, and targeted NGS. As we look at those, we will discuss what they are and when they should be used in food safety programs.
WGS | Shotgun Metagenomics | Targeted NGS | |
Type of DNA Sequencing | Non-targeted | Non-targeted | Targeted |
Testing Source | Pure isolate-based | Isolate independent | Isolate independent |
Turnaround Time | Several days | Less than a day to several days | Less than a day |
Cost | Expensive | Expensive | Same cost as PCR |
Sample Source | Limited to a pure isolate | Applicable to complex cultures | Applicable to complex cultures |
Detection Sensitivity | Sensitivity not applicable | Variable sensitivity | Highly sensitive |
Example Use Case | Traceback analyses | Broad investigative applications | Routine pathogen testing |
Whole-Genome Sequencing: Great for traceability studies
To start, let’s take a look at WGS. Most food safety professionals have probably heard of WGS, as it continues to grow in prominence. In short, WGS is the process of determining the complete DNA sequence of an organism. For a long time, only researchers and academics used WGS because it was time-intensive, costly, and needs special expertise to analyze the generated sequences. However, upon the introduction of NGS platforms, the cost of WGS has come down significantly. As a result, WGS has become routine in the clinical and food safety spaces.
WGS is a powerful tool for situations in which a lab needs detailed genetic characterization of a pure sample. By doing WGS of a given sample, the most detailed genetic footprint of that sample is created. This could include up to several million genetic building blocks per sample, for a bacterial sample. These genetic building blocks are then stitched together to create larger genetic scaffolds and then compared to a reference database to identify the microorganism being studied. Computer algorithms can notice the slightest differences between the DNA of the samples and that of the microorganisms in the database.
In the food safety space, WGS is not a good fit for routine pathogen testing because it is costly and slow and requires a pure culture. Instead, traceback analyses are prime candidates for WGS because this approach to NGS gives the resolution required to differentiate between strains and pinpoint where a food sample picked up, say, Salmonella Kentucky strain sequence type (ST) 198. Moreover, WGS is ideal for traceability studies because such studies rely on pure samples, which are prerequisites for WGS studies.
Traceback analyses aren’t the only use cases for WGS. Food safety scientists can use WGS whenever they need detailed characterization of a given genome and its functional attributes. For example, they can leverage WGS to determine which antibiotics an animal is resistant to and which nutrients they can metabolize.
Shotgun Metagenomics: Ideal for broad investigative applications
While WGS has decreased in cost, the application’s price point and turnaround time are not ideal for routine testing. What is more, the test requires pure cultures, which can be limiting. On the other hand, shotgun metagenomics, though expensive, does not have the same limitations on cultures. It can be run on pure or mixed microbial populations.
In shotgun metagenomics, DNA is randomly shredded into many small fragments that can be sequenced individually. Then, the sequences of these fragments are reassembled into their order, ultimately producing the complete sequence. Shotgun sequencing is unbiased and can generate unparalleled details about the identity of all genetic material within a biological sample. WGS is usually achieved by applying a shotgun approach to a pure sample. If the sample is complex, then applying shotgun sequencing generates shotgun metagenomics data.
A shotgun metagenomics approach to NGS is great for broad investigative projects like environmental monitoring studies, where food safety professionals need a complete view of the microorganisms in their lab and their functional attributes. Take, for example, biological monitoring programs. A shotgun approach can be used to analyze a bulk environmental water or soil samples looking for the presence or absence and function of indicator organisms, and machine-learning software can use the data to reconstruct the networks of ecological interactions. That way, food safety professionals can identify and minimize the risk of contamination.
There are some complicating factors with a shotgun approach. Imagine an environment with communities of bacteria with 10,000 different strains. However, the team only wants a detailed characterization of Listeria monocytogenes. It can be difficult to arrive at the right depth of information. Additionally, if the sample has a very high degree of complexity, the amount of data needed to confidently characterize the target microorganism could be very high, and by extension, the endeavor can become cost inefficient.
Targeted NGS: Built for routine pathogen testing
So far, we’ve discussed two non-targeted NGS applications. Now, let’s take a look at targeted NGS. Whereas WGS looks at the entire genome, targeted NGS looks at many loci /genetic markers within a broader pool of genetic materials. While this might sound like a limited PCR test, it’s different in a few fundamental ways. PCR-based tests only look at one genetic marker (or, in case of multiplex PCR, at a couple of genetic markers) rather than multiple genetic markers as is done in targeted NGS. As a result, the accuracy of PCR tests is lower than that of targeted NGS.
The other fundamental difference between PCR and a targeted sequencing-based approach is that PCR can only flag the presence and absence of a genetic marker. On the other hand, targeted DNA sequencing can read every single building block of a targeted region to make sure that the test amplified the correct target. Then, it can use algorithms to compare the sample’s building blocks with a reference database and confirm whether the target matches the DNA sequences of, say, Salmonella Saintpaul and not Salmonella Derby.
Another differentiation factor between PCR and targeted sequencing is the amount of information collected. Targeted sequencing can be utilized to increase the amount of information collected from a single bit (in case of PCR) by several orders of magnitude. Such high information content, beyond guaranteeing a superior performance, when combined with AI and machine learning can generate new layers of insight, like predictive analytics and analyzing trends that were not possible before. For example, targeted sequencing has the potential to lend more accuracy to shelf-life predictions.
PCR | Targeted NGS | |
Accuracy | Higher rates of false positives and negatives | >99.9% accuracy, resulting in dramatically lower rates of false positives and negatives |
Pathogen Profiling | Limited profiling with separate targeted serotyping usually available as a separate assay | Speciation, serotyping, and are faster, more inexpensive, and carried out simultaneously |
Throughput | Screen for one (or a couple) pathogen at a time | Screen for an array of pathogens in one test |
Insights | Binary yes/no answers | Professionals can choose the depth of information that they want |
To be sure, targeted NGS and PCR do share some commonalities. For starters, the turnaround time for detection is the same with both PCR and targeted NGS. In addition, both PCR and targeted NGS are far cheaper than both WGS and shotgun metagenomics. In fact, PCR and targeted NGS tests can have similar costs.
But with targeted NGS, scientists can do much more than they can do with a PCR test. Targeted NGS platforms are ideal for routine pathogen testing. Because of their targeted nature, these platforms can be fine-tuned to be very sensitive, detecting a needle in a haystack. Or, to put it in scientific terms, targeted NGS platforms can detect a specific serotype in a mixed sample, a pure sample, an individual colony, a liquid sample, or a solid sample. As a result, targeted NGS platforms are ideal for any application where accuracy is of the utmost importance.
Due to the superior accuracy of targeted NGS solutions, food manufacturers and labs can expect fewer ambiguous results that require lengthy confirmation steps. In that sense, targeted NGS testing can save companies both time and money by reducing their inventory hold times inherent to the confirmation process.
A powerful tool for food safety
NGS is a powerful resource in any food safety professional’s toolkit. Instead of simply detecting the presence of a specific microbe, scientists can gain greater insight into the microbial community in a food or environmental sample. As this technology takes hold, we can expect stronger, more sophisticated food safety programs to emerge.
Sasan Amini is the CEO and co-founder of Clear Labs. He holds a Ph.D. in genomics from Princeton University and spent three years at Illumina, the market leader in next-generation sequencing solutions.